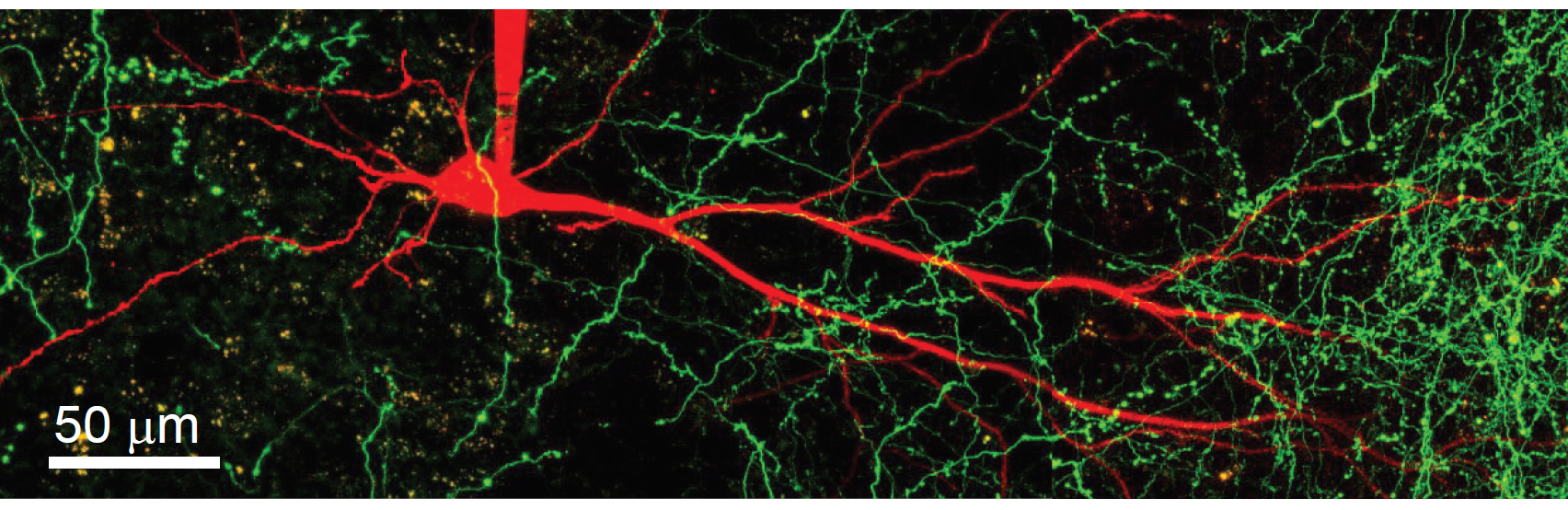
The main focus of the lab is to understand how the active, voltage dependent properties of dendrites and spines contribute to information processing on the single cell and network level during physiologically relevant learning-related behaviors.
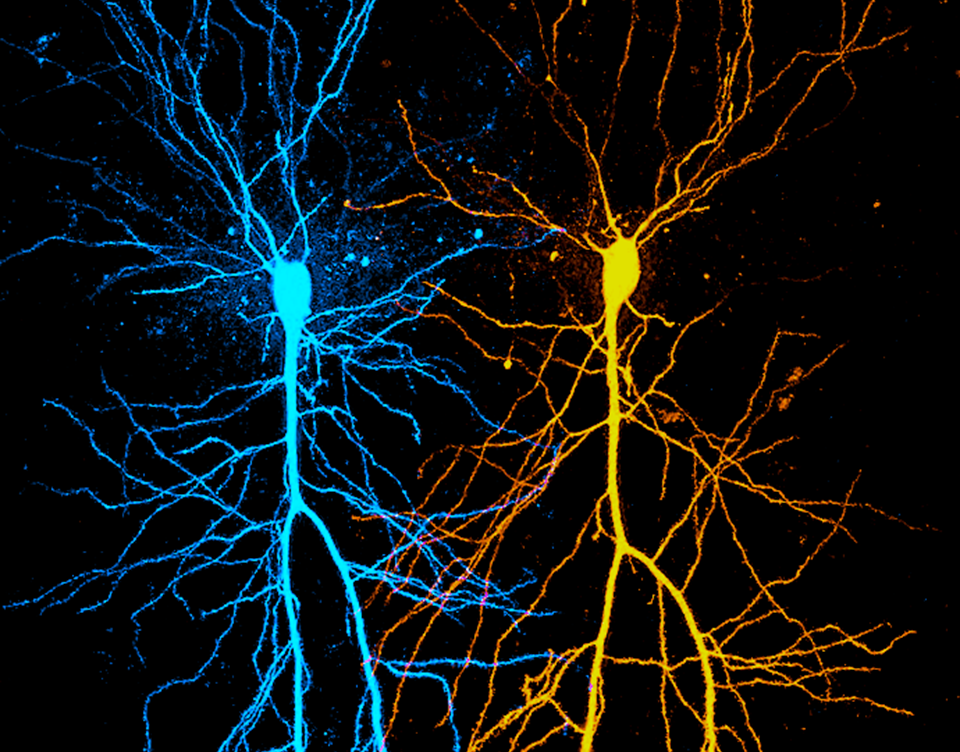
Most mammalian neurons receive thousands of excitatory synaptic contacts that are located on their elaborate dendritic tree. The passive and active electrical properties of dendrites thus have profound influence on the integration of synaptic inputs. Recent in vivo studies confirmed that in certain cortical neuron types active dendritic nonlinearities contribute to neuronal output. Understanding the molecular details of dendritic function is therefore essential to disentangle behaviorally relevant circuit computations.
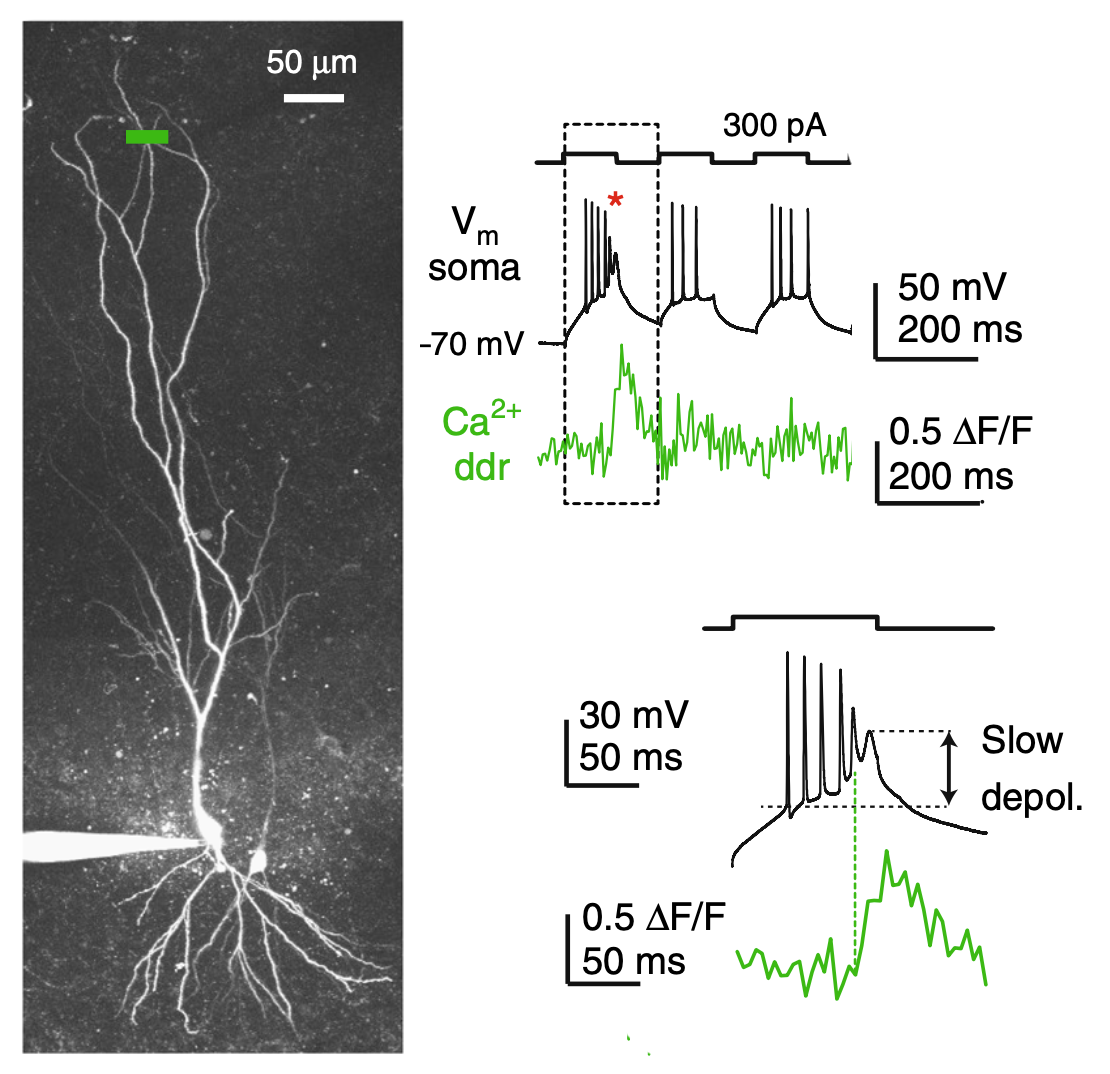
Voltage dependent ion conductances in dendrites and spines may generate local nonlinear interactions of voltage and Ca2+ signals arising from multiple spatiotemporally coactive synaptic inputs such as those provided by the correlated activity of a coherent neuronal ensemble. These mechanisms enable neurons to detect and transmit specific forms of information encoded by the activity of the upstream neuronal network, as well as to potentially reorganize synaptic strength depending on the actual parameters of incoming input patterns. Dynamic regulation of voltage dependent conductances enables dendritic plasticity mechanisms that may affect the precision, rate and timing of the output of neuronal populations receiving similar input patterns.
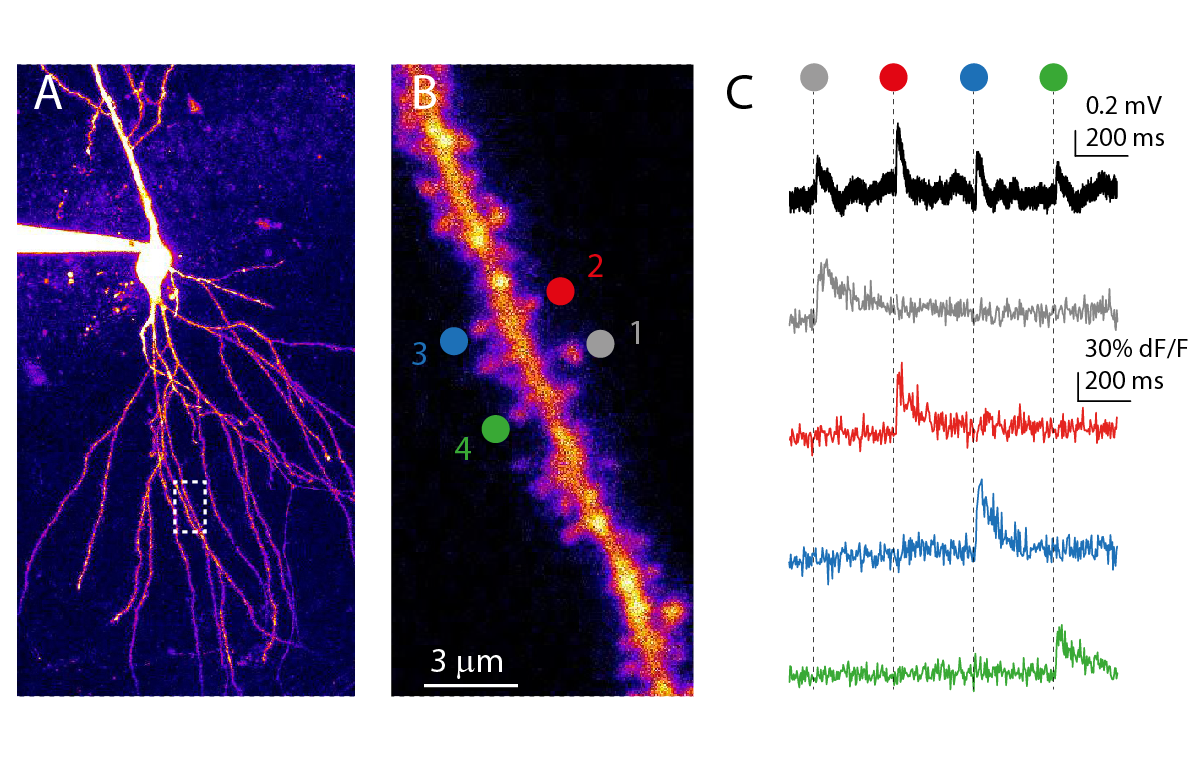
We use a combination of patch-clamp electrophysiology, two-photon imaging and two-photon glutamate uncaging to study these mechanisms in principal neurons of the the rodent hippocampal circuitry in vitro. We also investigate how dendritic activity is engaged during in vivo learning paradigms.
Our research is supported by the ERC, the Howard Hughes Medical Institute and the National Research, Development and Innovation Office of Hungary.